Electric Transformers
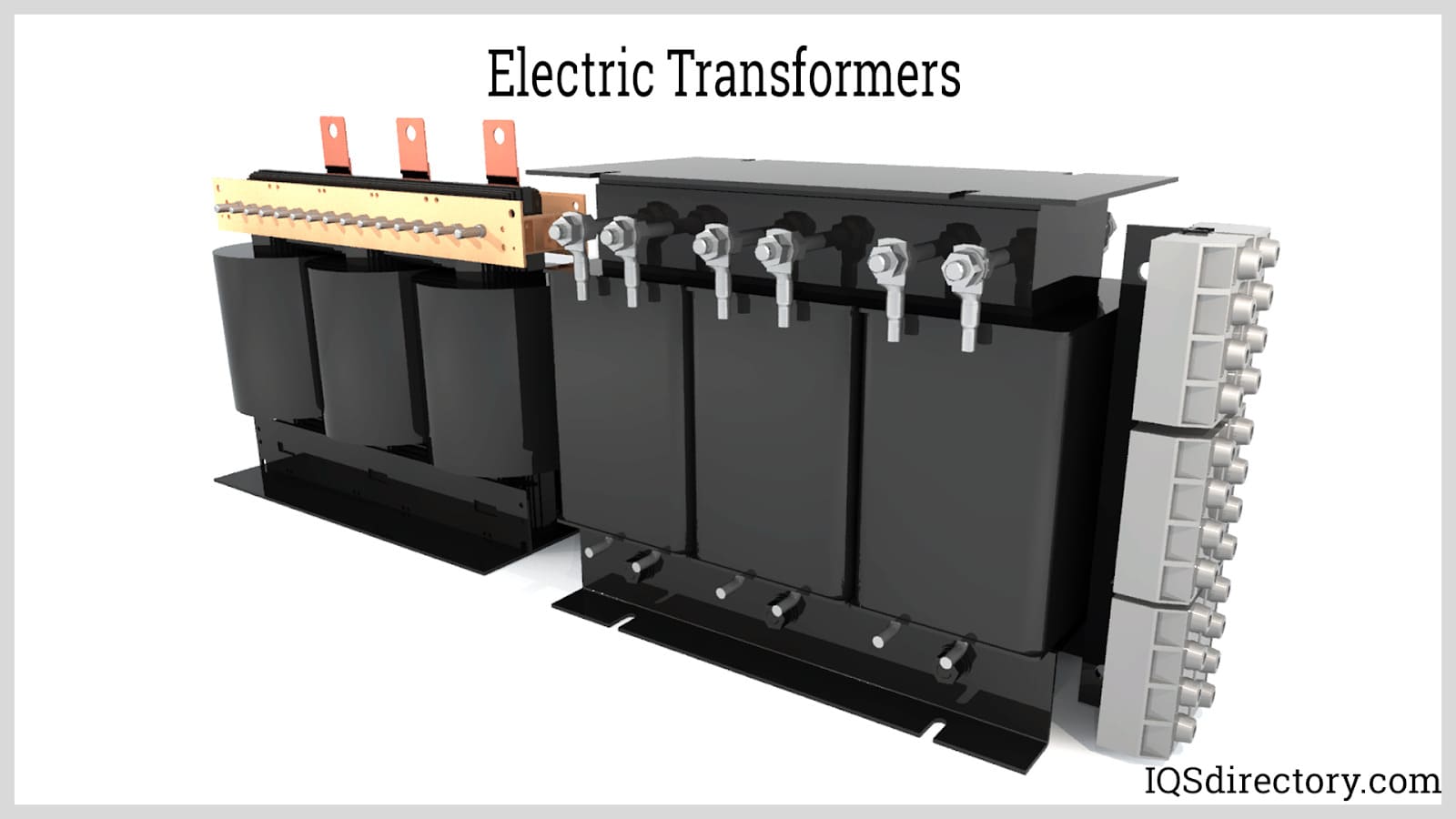
Electric transformers are static electrical machines that transform electric power from one circuit to the other without changing the frequency. An electrical transformer can increase or decrease the voltage with...
Please fill out the following form to submit a Request for Quote to any of the following companies listed on
This article offers an in-depth guide to toroidal transformers.
Read further to find out more.
A toroidal transformer is a type of electrical transformer constructed with a torus or donut-shaped core. Its primary and secondary windings are wound across the entire surface of the torus core, separated by an insulating material. This configuration minimizes the magnetic flux leakage. Therefore, a toroidal core is regarded as the ideal transformer core design.
Toroidal transformers are suitable for sensitive and critical electronic circuits because of several advantages over traditional square and rectangular-shaped transformers. Some of these advantages are high efficiency, quiet operation, minimal heat generation, and compact size. They are mostly seen in power supply systems, audio systems, control equipment, power inverters, and other electronic devices.
Before going into the specifics of toroidal transformers, it is best to first understand the basic operating principles of electrical transformers. An electrical transformer is a passive machine that transfers electrical energy from one circuit to another using a magnetic field to induce an electromotive force. This is done while the circuits being electrically isolated from each other. Transformers are used to increase (step-up) or decrease (step-down) voltages without changing the frequency of the electric current.
Electrical transformers operate based on Faraday‘s law of induction. This physical law states the relationship between the rate of change of a magnetic flux and the induced electromotive force. A magnetic flux is created when magnetic field lines pass through a conductor. It was observed that placing a conductor near a magnetic field with varying amplitude generates an electric current in that conductor.
The magnetic field involved in electromagnetic induction is typically from an electromagnet with a varying electric current. This varying electric current is commonly known as alternating current (AC). As the electrical current is generated and collapsed continuously at a given frequency, the magnetic field is also created and collapsed the same way. This magnetic field with varying amplitude induces an electric current to a second conductor. The induced electrical current in the second conductor has the same frequency as the electrical current from the electromagnet circuit.
A magnetic field with varying amplitude is not the only way to induce a current. A magnetic field can be imagined as a field with many lines of induction. Making the conductor "cut" through these magnetic field lines can generate an electric current. This phenomenon is seen in electrical generators.
The simplest transformer is a single-phase transformer. It has two electrical coils called primary and secondary windings. The primary winding is where the power supply is connected, while the secondary winding is where electricity is induced. These windings are wrapped in a closed-loop magnetic core.
The two windings are not electrically connected but are coupled by a magnetic field. Voltage can be increased or decreased in the secondary winding by altering the number of coils of a winding relative to the other. Since the transformer is considered a linear device, the voltage generated in the secondary winding can be predicted by determining the ratio of the number of turns of the windings. The result is known as the turns ratio (TR).
Simply put, power is obtained by multiplying voltage by the current. A transformer without any losses can be considered a constant power device, meaning the generated power in the secondary winding is the same as the power supply in the primary winding. Thus, to increase the voltage, the current must be decreased, and vice versa.
The common power rating of transformers ranges from 88 to 99%. Power losses are attributed to iron or core losses, copper loss, stray loss, and dielectric loss.
Core losses are from hysteresis or eddy currents. Hysteresis is due to the power required to reverse the magnetic field as it changes direction. This is given off as heat, which will then be dissipated from the machine. Eddy currents, on the other hand, are produced by the magnetic field from the primary winding within the core and are not part of the current generated for useful work. They are minimized by using laminated cores.
Copper loss is due to the resistance of the copper windings. All conductors have electrical resistances that cause a voltage drop as an electrical current passes through them. This loss cannot be easily reduced since it requires increasing the cross-section of the conductor. Consequently, this will require a larger and more expensive transformer. This loss causes a release in energy from the windings in the form of heat.
Stray loss is from the leakage of the magnetic field that influences other conductive parts of the transformer. Since this magnetic field is weak compared to what is present in the iron core, the eddy currents produced by stray magnetic fields cause negligible effect.
Transformer dielectric materials are the turn-to-turn or layer-to-layer insulation of the windings. Larger transformers also use transformer oil to insulate while preventing arcing and dissipating heat. Dielectric loss is caused by the degradation of the insulating materials and the transformer oil.
Transformer core shapes can be generally classified as solenoids and toroids. A solenoid is defined as a long, thin conductor that is coiled into tightly packed loops. Its conductor coils are helically wound in a straight direction. They are mostly seen in square and rectangular transformers. Common examples of solenoid cores are laminated EI and UI cores.
On the other hand, a toroid is similar to a solenoid but is constructed with a circular profile, creating a continuous structure. Toroid cores are specific to toroid transformers.
An ideal solenoid would be infinitely long with tightly packed coils, where each coil falls into a plane perpendicular to its central axis. In this solenoid, the generated magnetic field of the electric current is concentrated inside the solenoid coil. The intensity of the magnetic field outside the solenoid coil is theoretically zero.
However, this is not the case in an actual solenoid, whose length is always finite. Magnetic field lines always form closed loops. Because of this, some of the flux escapes at the ends of the solenoid to complete the loop. Without a conductive material to contain the magnetic flux, it can pass through other conductors outside the solenoid. This then contributes to the energy losses of the transformer.
To contain the magnetic flux in solenoid cores, additional material is used to create a return path. These additional paths, or limbs, contribute to the larger construction of the solenoid core.
Similar to an ideal solenoid, an ideal toroid would perfectly contain the magnetic flux. The magnetic flux density is concentrated inside the coil, while none is present in the outside regions. The ideal design of a toroid core is much more achievable than that of a solenoid. It does not need to be infinitely long since it is configured into a loop. The only requirement for the achieving a perfect design is to create windings that are uniformly and tightly wound to the whole toroid. This is likely why Faraday, when he discovered induction, used a toroidal core instead of a solenoid. Moreover, since a toroid is circular in construction, the magnetic flux easily forms a loop without requiring an additional return path.
The previous chapter discussed the fundamental differences between solenoid and toroid cores. By further analyzing these concepts, the benefits and limitations of both designs can be determined. Below are the advantages and disadvantages of toroidal transformers in comparison with other transformer types.
Transformer efficiency is the ratio of the output power of the secondary windings to the supplied power to the primary windings. Toroidal transformers have higher efficiency than other transformers, typically around 95 to 99%. This can be attributed to its near-ideal design, which perfectly contains the magnetic flux inside the windings. Thus, there is no leakage flux. Since the magnetic flux is highly concentrated inside the coil windings and the windings are evenly distributed to the whole toroid core, the magnetic flux is efficiently utilized to couple the primary and secondary windings.
Because of the effective containment of the magnetic flux, toroidal cores inherently shield adjacent components from electromagnetic interference (EMI). This characteristic makes them suitable for electronic devices with sensitive components. Transformers with solenoid cores are prone to leaking magnetic flux, additional shielding is used to prevent solenoid cores from creating EMI. The additional shielding only adds to their cost and size.
Minimal signal distortion is an advantage of transformers with low leakage flux. Leakage flux or stray magnetic fields can induce stray currents when their magnetic field lines cross nearby conductors. This allows unwanted signals to appear or interfere with other sensitive signals. This problem is highly evident in low-power circuits, where the signal can be easily distorted. That is why toroidal transformers are extensively used in audio systems, medical devices, measuring instruments, and power analyzers, where high signal resolution is required.
In single-phase transformers with EI and UI cores, additional material is added to its construction to provide a return path for the magnetic flux. EI cores have three limbs, only one of which is used for bearing the conductor coil. Thus, the core uses less than a third of its structure. For UI cores, there are two limbs used for carrying the coil, and both are used for carrying the coil, making the core utilization of UI cores higher. However, in this construction, the yoke is made larger than that of an EI core. Both the yokes of the EI and UI cores do not carry the conductor coils.
These problems are eliminated in toroidal cores. Transformers employing toroidal cores utilize the entirety of the core. There are no additional return paths to be added since the core is already looped in construction. This allows its construction to be much smaller than similarly rated EI and UI-cored transformers.
This advantage is a direct consequence of having a smaller core construction. Having a smaller core means fewer materials are used. The typical material for transformer cores is silicon steel. For more specialized applications, more expensive core materials and constructions are preferred because of their desirable characteristics, such as high permeability, low hysteresis, and low eddy current generation. Examples of these core materials are permalloys, cobalt-based amorphous alloys, and nanocrystalline materials.
Off-load losses are transformer losses caused by magnetizing the core even when there is no load connected to the secondary circuit. Transformers tend to consume power even in standby mode. Because of their efficient construction, core losses in a toroidal transformer are lower than in ordinary solenoid-core transformers. This means toroidal have fewer off-load losses.
Toroidal cores are constructed differently than solenoid cores; they have a continuous construction with virtually no air gap. Air gap contributes to different negative effects such as magnetic flux spreading, known as fringing, and noise. A common solenoid core such as an EI core has three air gaps. At these locations, magnetic flux leaks and passes through adjacent external components.
Transformer noise is due to mechanical humming generated by its core. It is produced when a core material vibrates and impacts adjacent core parts. Vibration is an effect produced by a phenomenon called magnetostriction. This is the changing of a material’s dimension upon magnetization.
Mechanical humming is more evident in transformer cores with loose components. EI and UI cores are typically constructed with laminated iron cores. These laminated cores can become loose over time, which causes them to vibrate and produce noise. Moreover, their structure is not continuous. They have air gaps between some of their limbs and yokes that can impact one another when vibrating. These problems are not present in toroidal transformers. Most toroidal cores do not use laminated cores and have a continuous structure with no air gaps.
This is another effect of having higher efficiency. Electrical energy losses in a transformer are eventually converted into vibrations and heat. Heat is generated mostly from eddy currents, hysteresis, and copper resistance. For larger transformers, an oil cooling system is used for dissipating heat. For transformers in small electronic devices, a metal heat sink is typically used. Because of the toroidal transformer‘s high efficiency, they generate less heat and can function even without cooling systems or heat sinks.
Although toroidal transformers have smaller profiles and use fewer materials, they are more expensive to make than other transformer types. This is due to the difficulty of winding the conductor coils around the toroid core. The process takes longer since each coil must be wound one at a time. The case is different with EI and UI coils. They are initially disassembled to their E and I piece. The limbs on which the coils are wound are easily accessible, enabling mass production.
Toroidal transformers are limited to single-phase applications. There are three-phase toroidal transformers available in the market, but they are less popular than the conventional core and shell-type transformers. Three-phase toroidal transformers are only used in special applications.
Three-phase toroidal transformers use three separate toroids, multiplying the cost of the transformer. This is not the case in core and shell-type transformers, where the coils can be wound on adjacent limbs. Core-types have three limbs, while shell-types have five. Their limbs have a common top and bottom yoke. Because of this configuration, their profile is greatly minimized instead of using three separate single-phase transformers.
Inrush current is the current spike when the transformer is turned on. This is due to the large initial current drawn by the primary circuit when there is a load connected to the secondary circuit. The magnitude of inrush current is greater in highly efficient transformers such as toroid transformers.
With toroidal transformers, inrush current can cause serious damage if not managed correctly. It can trip circuit breakers, cause fuses to blow, and produce complete failure of the transformer in more serious cases. The amount of inrush current determines the number of devices that can be connected to the power source.
Active or passive devices can be installed to protect from the effects of inrush current, which are chosen in accordance with the frequency of the inrush current as well as their performance, cost, and reliability. Passive inrush current limiters use a control mechanism such as an NTC thermistor, which works as an electrical resistor with high resistance at low temperatures.
Active devices are costly and may increase the size of the system. They are exceptionally sensitive and work by switching the high incoming current. Active devices protect electrical and mechanical systems by limiting inrush current.
A transformer core is made from magnetic materials. These materials can vary in levels of effective magnetic permeability, electrical resistivity, hysteresis, and so on. The efficiency of the transformer depends on the qualities of these magnetic materials, as the core contributes to most transformer losses. The common types of cores used in toroidal transformers are enumerated below.
Ferrite Cores: Ferrite cores are made from a metal-oxide ceramic, iron oxide, mixed with other metals such as cobalt, copper, nickel, manganese, and zinc. The two common types of ferrite cores are manganese-zinc ferrite and nickel-zinc ferrite cores. Compared to other types, ferrite cores have relatively low permeability, low saturation flux density, and low Curie temperature. Their biggest advantage is their high electrical resistivity, which helps reduce the generation of eddy currents.
Laminated Iron Alloy Cores: Laminated iron alloy cores are used in conventional transformers that operate at low to medium frequencies. They are made by rolling metal into flat sheets, which are stamped into the required shape of the core. The stamped and shaped sheets are placed in stacks, glued together, and compacted into a single solid piece. The layers of the compressed sheets are separated by insulation positioned between each of the sheets.
Laminated iron alloy cores are used in conventional transformers that operate at low to medium frequencies. They are made by rolling metal into flat sheets, which are stamped into the required shape of the core. The stamped and shaped sheets are placed in stacks, glued together, and compacted into a single solid piece. The layers of the compressed sheets are separated by insulation positioned between each of the sheets.
The lamination of laminated iron alloy cores reduces eddy currents, which are trapped in the thickness of each lamination. Silicon iron and nickel iron are two of the most used materials for manufacturing laminated cores.
Tape Wound Cores - In principle, tape wound cores are the same as laminated cores. They are more commonly used for making toroidal transformers than laminated cores. Instead of stacking ring-shaped laminations, tape wound cores are made from insulated metal ribbons wound into a spiral. It is then encapsulated by a thin sheet made from aluminum, or plastic.
The manufacturing process for tape wound cores is designed to produce transformer cores with the lowest loss and magnetizing currents. Modern methodology and technical advancements have eliminated the air gap at the face of tape wound cores, providing cores with very little hum and low exciting current.
Tape wound cores are used where thousands of transformers are needed, and weight and dimensions must be reduced. They are the most common core used for manufacturing toroidal transformers and are preferred over laminated cores due to their size.
Aside from specifying the type of transformer and core material, other specifications must also be given to complete the design. Below are some important transformer specifications that are included in the product datasheet.
Number of Primary and Secondary Windings: A simple toroidal transformer has only one primary and one secondary winding. More windings can be addede for better flexibility. A usual design, there is one primary and two secondary windings. These designs have six lead wires. The secondary windings are used independently and can be configured to be in parallel or series.
Electric transformers are static electrical machines that transform electric power from one circuit to the other without changing the frequency. An electrical transformer can increase or decrease the voltage with...
An isolation transformer, just like typical transformers, is a non-moving device that transmits electrical energy from one circuit to another without requiring any physical contact. It works on the idea of magnetic...
Power transformers are electrical instruments used in transmitting electrical power from one circuit to another without changing the frequency. They operate by the principle of electromagnetic induction. They are used in transmitting electrical power between...
An electrical transformer is a passive machine that transfers electrical energy from one circuit to another using a magnetic flux to induce an electromotive force. Transformers are used to increase (step-up) or decrease (step-down) voltages without changing the frequency of the electric current...
Electronically operated equipment depends on power transformers to convert electrical currents into voltage. Current transformers store and transport energy through power lines and grids...
Power supplies are electrical circuits and devices that are designed to convert mains power or electricity from any electric source to specific values of voltage and current for the target device...
An AC power supply is a type of power supply used to supply alternating current (AC) power to a load. The power input may be in an AC or DC form. The power supplied from wall outlets (mains supply) and...
An AC power cord is a detachable way of providing an alternating current of electric energy from a mains power supply to an electrical appliance or equipment. Serving industries like...
A coil spring is an elastic element made of metal or heavy plastic in the form of curls or ringlets of round wire or cord that is wrapped around a cylinder. The winding of a coil spring can be loose or tight in a helical shape depending on the application for which it is made...
A DC DC power supply (also known as DC DC Converter) is a kind of DC power supply that uses DC voltage as input instead of AC/DC power supplies that rely on AC mains supply voltage as an input...
A DC power supply is a type of power supply that gives direct current (DC) voltage to power a device. Because DC power supply is commonly used on an engineer‘s or technician‘s bench for a ton of power tests...
An electric coil, or electromagnetic coil, is an electrical conductor that contains a series of conductive wires wrapped around a ferromagnetic core that is cylindrical, toroidal, or disk-like. Electric coils are one of the simplest forms of electronic components and provide...
Electrical plugs, commonly known as power plugs, are devices responsible for supplying and drawing current from a receptacle to the circuitry of an electrical appliance...
By definition a power supply is a device that is designed to supply electric power to an electrical load. An electrical load refers to an electrical device that uses up electric power. Such a device can be anything from...
Inductors are passive two terminal components of an electric or electronic circuit that are capable of storing energy in magnetic form. They oppose sudden changes in current and they are also called coils or chokes...
A NEMA connector is a method for connecting electronic devices to power outlets. They can carry alternating current (AC) or direct current (DC). AC current is the typical current found in homes, offices, stores, or businesses...
A power cord is an electrical component used for connecting appliances to an electrical utility or power supply. It is made from an insulated electrical cable with one or both ends molded with connectors...
A programmable power supply is a method for controlling output voltage using an analog or digitally controlled signal using a keypad or rotary switch from the front panel of the power supply...
A solenoid coil is a common electrical component that uses a wire that is tightly wrapped around a core, usually made of metal, to generate an electromagnetic field. When an electrical current is passed through the coil, the electromagnetic field that is created provides energy for linear motion...
A voice coil is a winding of a wire, usually copper, aluminum, or copper-clad aluminum that is wrapped around a former (often called a bobbin) and then attached to the apex of a speaker cone...
Thomas Edison developed the power distribution system in 1882. He wrapped a copper rod in jute, a soft shiny fiber from plants, as an insulator. The jute wrapped copper rod was placed in a pipe with a bituminous compound...