Electric Transformers
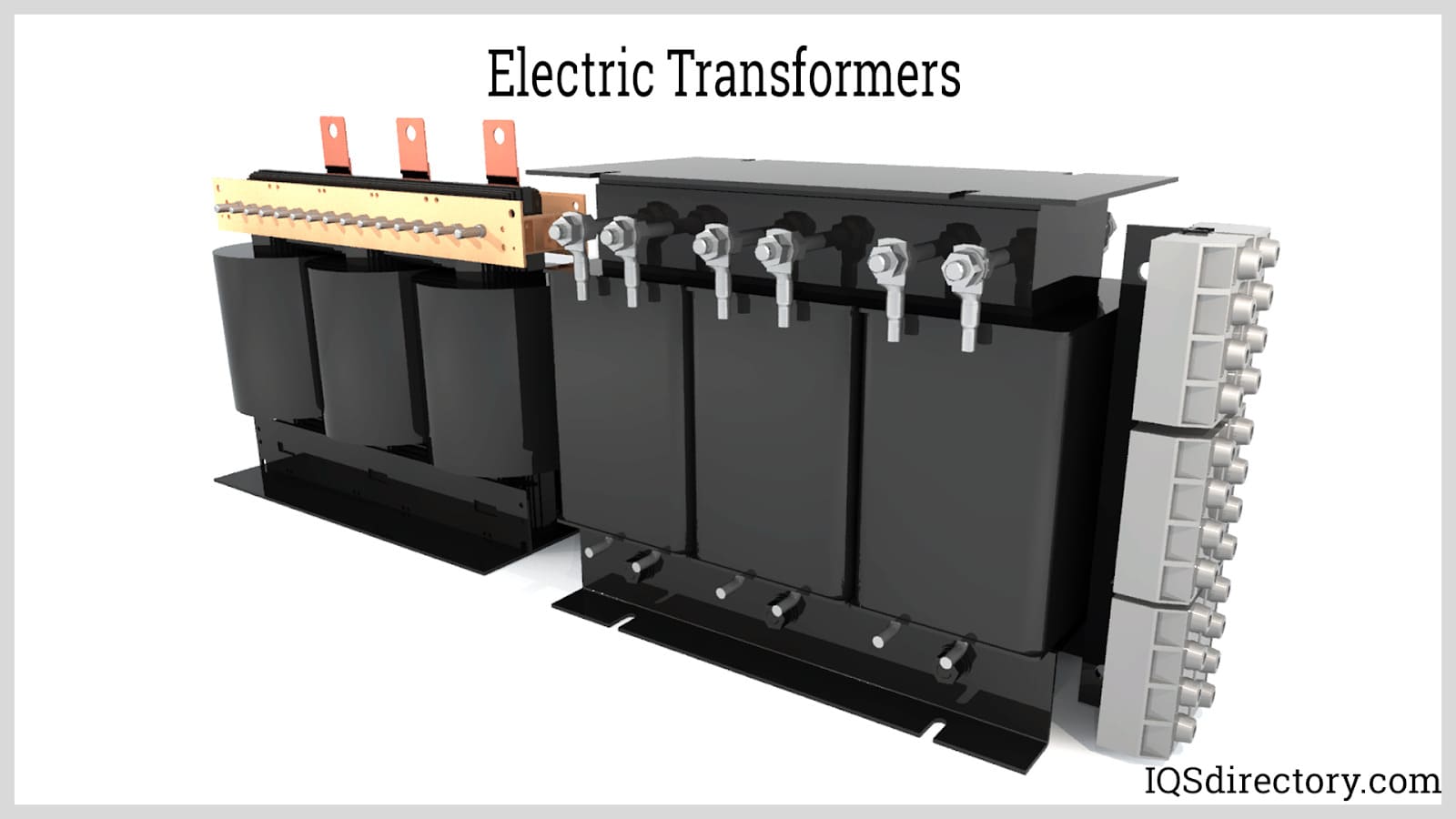
Electric transformers are static electrical machines that transform electric power from one circuit to the other without changing the frequency. An electrical transformer can increase or decrease the voltage with...
Please fill out the following form to submit a Request for Quote to any of the following companies listed on
This article provides a complete guide to the operating principles, configurations, and constructions of a three-phase transformer.
An electrical transformer is a passive machine that transfers electrical energy from one circuit to another using a magnetic flux to induce an electromotive force. Transformers are used to increase (step-up) or decrease (step-down) voltages without changing the frequency of the electric current. Also, since the two circuits or coils are electrically isolated from each other, transformers can be used for isolation. This is particularly useful for signal processing circuits.
Electrical transformers operate based on Faraday‘s law of induction, which states the relationship between the rate of change of a magnetic flux and the induced electrical field. It was observed that placing a conductor near a varying magnetic field creates an electric current in that conductor. The magnetic field can be from an electromagnet with a varying electric current, such as an alternating current (AC). This electromagnetic circuit is referred to as the primary winding. As the electrical current is generated and collapsed continuously at a given frequency, a magnetic field is also created and collapsed the same way. This magnetic field with varying amplitude induces an electric current to conductors influenced by its flux. The influenced conductor is usually a coil, referred to as the secondary winding. The induced electrical current has the same frequency as the electrical current from the primary circuit.
A magnetic field with varying amplitude is not the only way to induce a current. A magnetic field can be imagined as a field with many lines of induction. Making the conductor "cut" through these magnetic field lines can cause an electric current to be generated. This phenomenon is seen in electrical generators.
As mentioned earlier, transformers are used to change voltage levels as needed. To understand three-phase transformers, it is best to understand first the construction of a single-phase transformer. A single-phase transformer has two electrical coils as, again, the primary and secondary winding. The primary winding is where the power supply is connected, while the secondary winding is where electricity is induced.
The two windings are not in contact with each other but are connected by a closed magnetic iron core. It provides a path for the magnetic field to flow. Since the magnetic field is flowing through it and it is also a conductor, electrical currents are generated within. These circulating currents, known as eddy currents, cause energy losses and heating in the transformer. The eddy currents are reduced by increasing the resistivity of the core by using thin sheets or laminations.
The two windings are not electrically connected but linked by a magnetic field. Voltage can be increased or decreased in the secondary winding by altering the number of coils of a winding relative to the other. Since the transformer is considered a linear device, the voltage generated in the secondary winding can be predicted by determining the ratio of the number of turns of the windings. This is known as the turns ratio (TR). The ratio of the primary and secondary voltage is equal to the turns ratio.
Simply put, power is obtained by multiplying voltage by the current. A transformer without any losses can be considered a constant power device, meaning the generated power in the secondary winding is the same as the power supply in the primary winding. Thus, to increase the voltage, the current must be decreased, and vice versa.
Common power ratings of transformers range from 88 to 99%. Power losses are attributed to iron or core losses, copper loss, stray loss, and dielectric loss. Core losses are from hysteresis and eddy currents. Hysteresis is due to the required power to reverse the magnetic field as it changes direction. This is given off as heat which will then be dissipated from the machine. Eddy currents, on the other hand, are produced by the magnetic field from the primary winding within the core and are not part of the current generated for useful work. As described above, eddy currents are minimized by using laminations.
Copper loss is due to the resistance of the copper windings. All conductors have electrical resistances, which cause a voltage drop as an electrical current passes through them. This loss cannot be easily reduced since it requires increasing the cross-section of the conductor. Consequently, this will require a larger and more expensive transformer. This loss causes a release of energy from the windings in the form of heat.
Stray loss is from the leakage of the magnetic field that influences other conductive parts of the transformer. Since this magnetic field is weak compared to what is present in the iron core, the eddy currents produced cause negligible effect.
Transformer dielectric materials are the turn-to-turn or layer-to-layer insulation of the windings. The transformer oil, on the other hand, is used to insulate, prevent arcing, and dissipate heat. Dielectric loss is caused by the degradation of the insulating materials and the transformer oil.
Three-phase transformers are transformers that operate with a three-phase electrical system. The type of transformer described in the previous chapter is a simple single-phase transformer. The working principle of three-phase transformers is the same, Faraday‘s Law of Induction. Single-phase and three-phase transformers differ in wiring configurations. To further understand, it is better to take a look at three-phase electrical systems.
Single-phase and three-phase electrical systems use alternating current (AC). AC is a form of electricity that is constantly changing amplitude and direction, usually characterized by a sine wave. However, other waveforms can be created, such as complex, triangular, and square waves. AC signals have three main properties: period, frequency, and amplitude. Period and frequency describe the time component of the wave, while amplitude describes the strength or magnitude of the electricity.
One complete AC waveform has a peak and a trough. For a 360° cycle, this occurs at 90° and 270°. A single-phase electrical system has a current with one peak and one through flowing on a single conductor. At these points, the amplitude is greatest but in different directions. For three-phase systems, the current has three peaks and three troughs flowing on three separate conductors. These currents are out of phase by 120° from each other. In three-phase electrical systems, it can be observed that the greatest amplitude is reached more frequently for a given period. This helps deliver power at an almost constant rate.
A three-phase transformer consists of six windings, three for the primary and three for the secondary. The windings on each side (primary and secondary side) can be connected in either delta or star configurations. These windings can be viewed as separate single-phase windings. In theory, three single-phase transformers can be connected, creating a three-phase transformer.
The two main forms of three-phase connections are delta and star. The delta connection, also known as mesh connection, consists of three windings connected at the ends of each other, forming a closed loop. These ends are connected to a terminal. It can be observed that there is no neutral point. Grounding connections are used instead. Delta connections can also be configured as high-leg systems by grounding the center point of one phase. In this configuration, the voltage measured across the line opposite to the center-tapped phase and ground is higher than measured across terminals.
The star connection, also known as the wye connection, consists of three windings and four terminals. One end of the three windings is connected to a common neutral point or terminal, while the others form the three phases of the circuit.
Using either delta or star connections has its advantages and disadvantages. To further understand, it is best to know the difference between phase and line voltages and current. Phase voltage and current are measured across a single component. The line voltage and current, on the other hand, are measured across two terminals. Phase and line relationships of delta and star connections are summarized below.
Delta | Star | |
Voltage | VL = VP | VL = VP x √3 |
Current | IL = IP x √3 | IL = IP |
Where: VL = Line Voltage
VP = Phase Voltage
IL = Line Current
IP = Phase Current
In star connections, the line and phase currents are the same, whhile voltages differ by a factor of √3. Hence, it is possible to supply power with different voltages without the need for additional transformers. For example, a 230V star circuit can also supply 400V. The 230V supply can be obtained by connecting from a phase terminal and neutral, while the 400V is from connecting two phases.
For delta connections, the line and phase voltages are the same, but the currents differ by a factor of √3. The line current of a delta connection is larger than the phase current. Because of this, to supply a given power, thicker insulation is required. Star connections are preferable to use for power transmission and distribution networks since they use less insulation material.
An advantage of delta connections is reliability. If one winding of the primary side fails, it is still possible for the secondary side to supply the same voltage provided that the remaining two phases can carry the load.
In a three-phase transformer, the primary and secondary sides can either have the same or different configurations. There are four permutations possible: star-star, star-delta, delta-star, and delta-delta.
This type has both star windings on the primary and secondary sides. Line voltages on each side are √3 times the voltage of a single phase. The main advantage of a star-star connection is the access to the neutral terminal on both sides of the transformer which can be grounded if desirrhe grouf,ed. By connecting the star neutral to the ground, distortion to the waveform is eliminated. Without grounding, this configuration‘s operation is satisfactory only if the loads on al three phases are balanced.
From the name itself, this type has the primary side in star connection while the secondary side is in delta. By having a star connection at the primary side, the star‘s neutral point can be connected to ground to avoid distortion. Also, the phase voltage of the primary is equal to the supply line voltage divided by √3, or only about 58%. Thus, for a 1:1 turns ratio, a star-delta connection will provide reduced voltage to the secondary side by a factor of 0.58. This makes the star-delta connection applicable to step-down transformers.
The main disadvantage of this configuration is that the primary side voltages are from line to neutral, while the secondary line voltages are from line-to-line. The primary is then out of phase with the secondary side. This makes it difficult to parallel star-delta transformers with other types of winding connections.
On the primary side, the line and phase voltages are the same, while on the secondary side, the line voltage is equal to the phase voltage multiplied by √3. Thus, for a 1:1 turns ratio, the secondary line will provide an increased voltage by a factor of √3. Delta-star transformers are used for step-up applications. Another application of this type is distribution systems. Since the secondary side has a neutral, it can also serve as a single-phase power supply aside from supplying the full three-phase.
Like the star-delta, by having different windings of the primary and secondary sides, the resulting electricity is out of phase.
In this configuration, the line-to-line voltages on both the primary and secondary sides are equal to the phase voltage. The main advantage of this type is that even under unbalanced loads, the three-phase voltages remain equal. Also, since both sides have the same type of windings, there is no phase shift between them.
The disadvantages of delta-delta connections are the absence of a neutral connection on both sides and the more expensive coils required. Delta connected transformers must be wound to the full line voltage. This makes this type useful for low voltage applications.
There are other types of three-phase transformers aside from these four permutations. The other types are a result of modifying the basic star and delta windings. The other types are as follows.
Open delta connection, also known as V-V, is achieved by removing two matching windings from the primary and secondary sides of a delta-delta circuit. In this connection, three equal three-phase voltages are available at the secondary terminals at no load. This is usually done when the three-phase load is too small for the installation of a full three-phase transformer. For a given open delta transformer bank, the three-phase load that can be carried without exceeding the ratings of the transformers is only 58% of the original load. In case the load is increased, the open delta may be closed, making it a delta-delta connection.
This is also known as a T-T connection. This is accomplished by having two transformers, with one transformer having center taps, on both the primary and secondary winding. The main transformer has the center taps while the other transformer has a 0.87 tap known as the teaser transformer. The full rating of the transformer is not being utilized since the teaser transformer only operates at 87% of its rated voltage. The winding is represented by the figure below. This type of connection is done to link a three-phase system with a two-phase system. A common application of this conversion is a power supply on a two-phase system electric furnace.
A high leg delta is done by center tapping the delta connected secondary side and connecting this center tap to ground. This configuration results in a three-phase, delta connected supply, and a single-phase supply. This is mostly used in residential and commercial distribution systems where consumers are supplied with 240V line-to-line for large equipment or 120V line to neutral for lights and appliances without the need for an additional transformer.
High leg delta connection transformers are also known as red leg connections, wild leg connections, or orange leg connections. The normal use of high leg delta connections is found in North America, with it mainly being used in the United States. The orange leg high leg delta connection is in reference to a 208V supply, which is marked with an orange outer finish.
Normal transformers are loud and lack energy efficiency. Toroidal transformers break with tradition, operate very quietly, and produce minimal heat. The core of a toroidal transformer is shaped like a donut with primary and secondary windings that are separated by insulation, a design that minimizes magnetic leakage that causes noise.
The power of a toroidal transformer is stepped up or down in accordance with the magnetic fields passing through the secondary coil. The quantity of voltage is determined by the number of windings on the secondary coil with a ratio of two to one of the windings doubling the voltage. The primary coil creates a positive magnetic field that allows electrons to easily flow in either direction.
With an alternating current (AC) current signal of a toroidal transformer, the magnetic field reaches a peak and falls to zero voltage, which completes the first half cycle. After the completion of the first cycle, the electrons move in an opposite direction through the coil as a negative magnetic field. The switch in the direction of the magnetic field brings the voltage back to zero to complete a full cycle.
The noise level of a toroidal transformer is far less than other transformers, which is due to the size of the magnetic field they generate. The donut shape of the core makes it possible to have very tight windings that are unable to vibrate. This lack of vibration eliminates the possibility of noise and makes a toroidal transformer very secure.
The previous chapter discussed the types of three-phase transformers according to their phase windings. Three-phase transformers can also be classified with respect to their construction. Construction of three-phase transformers can be done by creating a single core with the primary and secondary windings combined or by connecting three single-phase transformers.
In a core-type transformer, the windings are evenly split and wound on the limbs of the core. The core consists of three limbs on the same plane. Each of these limbs contains both the primary and secondary windings. These windings may be better referred to as the high voltage and low voltage windings. The low voltage windings are wound closest to the core since it is easier to insulate. The high voltage coil is then wrapped around the low voltage winding with insulation between them. In this construction, the windings are magnetically coupled with each other, where one winding uses the other two limbs as a return path for its magnetic flux.
The shell-type transformer can be viewed as three separate single-phase transformers since the magnetic fields of the three phases are almost independent of each other. This type of transformer has a core with five limbs. The high voltage and low voltage windings are wrapped around the three main limbs, similar to the core-type, where the low voltage is closest to the core. By having the two outer limbs, the magnetic flux has additional return paths. As the magnetic field comes to the yoke, the magnetic flux divides into two. Thus, the yoke and the outer limbs can be sized as half of the main limbs. By decreasing the size of the yoke, the overall height of the transformer can also be decreased.
In the previous chapter, the windings and cores are discussed in detail. Other parts are just as important. This chapter mentions the other parts of a three-phase transformer.
Insulations act as a barrier system and are used to separate the windings from the core and the two windings from each other. There are several types of insulations that are used in transformers, which include oil, paper, tape, pressboard, and laminated wood.
There are two functions of the transformer oil: cooling and insulation. Since the core and windings generate heat, oil is used to transport and expel this heat. That is why transformer oils have high heat capacities. Oil flow can be made either by the thermosiphon effect or by pumping. Oil also has insulation properties preventing arcing and shorting.
The tank protects the cores and the windings from the outside environment. It also acts as a container for the oil. The tank is evacuated of air and other materials that may contaminate the oil and compromise the insulations‘ dielectric properties.
Heating the oil causes it to expand. A separate vessel from the tank is installed, known as the conservator.
As the oil absorbs heat from the system, it then transports this heat to the cooling system. The cooling system collects hot oil, cools it through the air- or water-cooled tubes then returns it to the windings and core. Below are the designations of transformer cooling systems as standardized by IEC.
These are used to vary the output voltage. During loaded conditions, the output voltage of the transformer may decrease. Thus, there is a need to adjust the voltage ratio by modifying the tapping turns. The adjustment is made by using a tap changer. The type of tap changer depends on the frequency with which it is required to change the output voltage.
Also known as the Buchholz relay, this part collects free gas bubbles from the transformer tank. The presence of free gas indicates a fault within the transformer.
These are used to monitor oil temperature.
These are used to remove moisture from the air space above the oil level of the conservator. This maintains the dryness of the transformer oil.
These are safety devices used to relieve overpressure in the event of oil flashing due to short circuits.
Electric transformers are static electrical machines that transform electric power from one circuit to the other without changing the frequency. An electrical transformer can increase or decrease the voltage with...
An isolation transformer, just like typical transformers, is a non-moving device that transmits electrical energy from one circuit to another without requiring any physical contact. It works on the idea of magnetic...
Power transformers are electrical instruments used in transmitting electrical power from one circuit to another without changing the frequency. They operate by the principle of electromagnetic induction. They are used in transmitting electrical power between...
A toroidal transformer is a type of electrical transformer constructed with a torus or donut-shaped core. Its primary and secondary windings are wound across the entire surface of the torus core separated by an insulating material...
Electronically operated equipment depends on power transformers to convert electrical currents into voltage. Current transformers store and transport energy through power lines and grids...
Power supplies are electrical circuits and devices that are designed to convert mains power or electricity from any electric source to specific values of voltage and current for the target device...
An AC power supply is a type of power supply used to supply alternating current (AC) power to a load. The power input may be in an AC or DC form. The power supplied from wall outlets (mains supply) and...
An AC power cord is a detachable way of providing an alternating current of electric energy from a mains power supply to an electrical appliance or equipment. Serving industries like...
A coil spring is an elastic element made of metal or heavy plastic in the form of curls or ringlets of round wire or cord that is wrapped around a cylinder. The winding of a coil spring can be loose or tight in a helical shape depending on the application for which it is made...
A DC DC power supply (also known as DC DC Converter) is a kind of DC power supply that uses DC voltage as input instead of AC/DC power supplies that rely on AC mains supply voltage as an input...
A DC power supply is a type of power supply that gives direct current (DC) voltage to power a device. Because DC power supply is commonly used on an engineer‘s or technician‘s bench for a ton of power tests...
An electric coil, or electromagnetic coil, is an electrical conductor that contains a series of conductive wires wrapped around a ferromagnetic core that is cylindrical, toroidal, or disk-like. Electric coils are one of the simplest forms of electronic components and provide...
Electrical plugs, commonly known as power plugs, are devices responsible for supplying and drawing current from a receptacle to the circuitry of an electrical appliance...
By definition a power supply is a device that is designed to supply electric power to an electrical load. An electrical load refers to an electrical device that uses up electric power. Such a device can be anything from...
Inductors are passive two terminal components of an electric or electronic circuit that are capable of storing energy in magnetic form. They oppose sudden changes in current and they are also called coils or chokes...
A NEMA connector is a method for connecting electronic devices to power outlets. They can carry alternating current (AC) or direct current (DC). AC current is the typical current found in homes, offices, stores, or businesses...
A power cord is an electrical component used for connecting appliances to an electrical utility or power supply. It is made from an insulated electrical cable with one or both ends molded with connectors...
A programmable power supply is a method for controlling output voltage using an analog or digitally controlled signal using a keypad or rotary switch from the front panel of the power supply...
A solenoid coil is a common electrical component that uses a wire that is tightly wrapped around a core, usually made of metal, to generate an electromagnetic field. When an electrical current is passed through the coil, the electromagnetic field that is created provides energy for linear motion...
A voice coil is a winding of a wire, usually copper, aluminum, or copper-clad aluminum that is wrapped around a former (often called a bobbin) and then attached to the apex of a speaker cone...
Thomas Edison developed the power distribution system in 1882. He wrapped a copper rod in jute, a soft shiny fiber from plants, as an insulator. The jute wrapped copper rod was placed in a pipe with a bituminous compound...